An indispensable cross-industry discipline for advancing product design and manufacturing, materials science is a field defined by innovation and creative thinking. Its focus is on how materials behave at every level, including the way in which microstructures and the arrangement of individual atoms and molecules can have significantly influence a material’s behavior- and how a structure can be manipulated to influence desired performance.
Most metal additive manufactured parts are made from alloys — both conventional and novel. However, there are very few alloy parts produced this way that have the same quality or retain the same mechanical properties offered by similar alloys when manufactured via a conventional process such as forging. Their behavior does not respond in the same fashion, largely due to the rapid melting and solidification they undergo in the additive manufacturing process. As the additive manufacturing industry has faced a challenge in retaining or even improving the characteristics of metal alloys, it has looked to materials science and engineering to enable the development of alloy parts with superior mechanical properties.
Influencing a material’s microstructure, in particular, can lead to some remarkable behaviors including a material’s flexibility — how far the material can be stretched or bent. It can also impact the hardness of a material, make it more corrosion resistant, or enhance its ability to conduct electricity or heat.
A perfect example is shape memory alloys — or metals that can be bent, then returned to their original shape by applying heat.
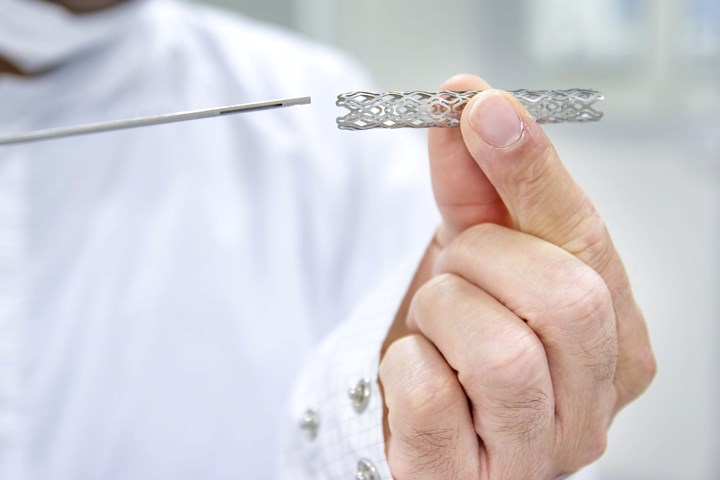
Nitinol’s shape memory properties make it an excellent material for minimally invasive medical devices such as stents. Photo Credit: Shutterstock
Nitinol and Its Applications
Discovered over 50 years ago, nitinol (NiTi) is an especially valuable metal alloy that has revolutionized numerous industries. Made of 50% atomic nickel and 50% atomic titanium, it has unique properties, allowing for its superelasticity and “shape memory effect,” meaning it can change shape depending on temperature. This special behavior is now seeing nitinol increasingly used in the medical, dental and aerospace industries.
Nitinol is an excellent material to use when creating components for minimally invasive medical devices, such as guidewires, catheters and stents. When medical professionals need to navigate in particularly tight areas, nitinol has both the flexibility to change shape as needed and the durability to endure high amounts of strain.
Read More: 3D Printed Nitinol Opens New Possibilities for Arterial Stents
Stents that are used to keep arteries open are perhaps the clearest example of the superelasticity and the shape memory effect of nitinol are so beneficial. When stents are inserted into the body, they can be compressed down to tiny size to be used in minimally invasive procedures. When they are placed at the right point in the artery, however, they expand to fill the necessary space and brace the inside arterial wall, a procedure that could not be done using stainless steel.
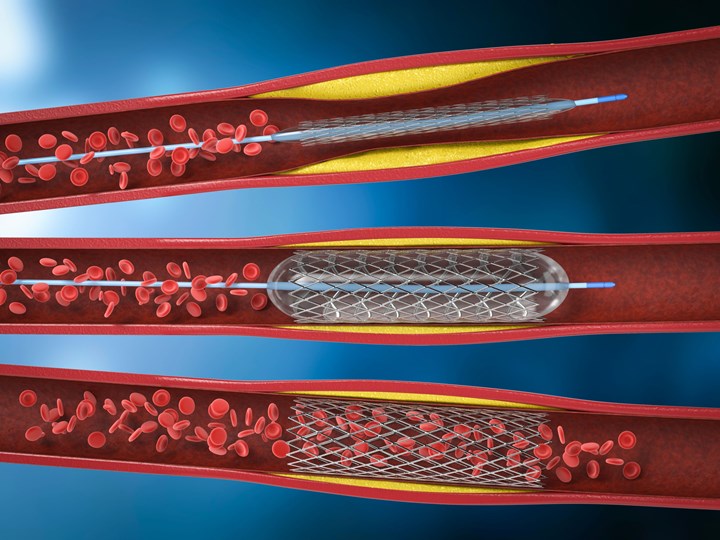
When nitinol stents are placed in the artery, they return to their original, “remembered” size, expanding to fill the necessary space. Photo Credit: Shutterstock
In orthopedic procedures, surgeons need components that will help patients regain flexibility and range of motion, as well as easily adapting to an individual patient’s tissue. Nitinol is the preferred material for these components, as it mimics bone mechanical behavior. Orthodontists also need wires and brackets that hold braces together and perform the function of moving teeth, so nitinol’s shape memory is particularly useful here as well.
Manufacturing Challenges with NiTi
Despite all its advantages, developing components made from nitinol can have its challenges. The alloy can be difficult to machine, so product design has typically been limited to simple structures. Such difficulties include high toughness, high ductility and work hardening in cutting processes. Traditional machining results in excessive tool wear, high cutting forces and surface degradation, often ending in low workpiece quality with inferior chip breaking and burr formation.
And while additive manufacturing can offer improved production efficiency and greater design freedom, there are still issues to be overcome when 3D printing with nitinol.
Vaporization of the nickel during the additive manufacturing process can lead to a decrease in the nickel-titanium ratio, thereby increasing the transformation temperature. Additionally, oxygen pickup inside the material can also affect the transformation temperature, negatively impacting shape memory and affecting the overall performance of the intended application.
Maintaining Atmospheric Conditions for 3D Printing
Even extremely small variations in oxygen content can impair the mechanical or chemical properties of metals and alloys sensitive to oxygen — including nitinol — and can affect the composition of the end product, resulting in negative physical characteristics such as discoloration and even poor fatigue resistance.
Monitoring and maintaining low levels of O2 inside of metal 3D printers alleviates challenges with printing materials such as nitinol that are prone to oxygen pickup. Photo Credit: Shutterstock
For the printing of nitinol components in particular, it is critical to maintain both a low and constant O2 level. If this is not achieved, the material can oxidize — either from too high an O2 level or the mechanical properties varying from one part to the next.
Although the atmosphere in the 3D printing chamber is typically purged with high-purity argon to rid it of oxygen, impurities may still remain present due to incomplete purging and small leakages. Typically, the level of residual oxygen after purging is around 1,000 parts per million (ppm) — far off the ideal of less than 10 ppm.
The materials scientists and engineers at Linde have dedicated the past few years to developing technology to overcome these atmospheric impurities in order to give manufacturers optimal printing conditions. The result, ADDvance O2 precision, provides continuous analysis of the gas atmosphere, detecting oxygen levels with high precision without cross-sensitivity. Recognizing O2 concentrations as low as 10 ppm, the unit automatically initiates a purging process to maintain the atmosphere as pure as needed.
Unique and bespoke gas mixtures have also been developed to help maintain printer conditions, such as ADDvance Laser230, a combination of argon and helium developed specifically to optimize printing outcomes. Successfully tested on a range of alloys including high-strength aluminum AISi10MG, nickel-based superalloys, titanium, nitinol and stainless steel, the gas mixture was found to reduce particle redeposition by up to 30% and powder loss by up to 20%. It also saves on maintenance time, with fewer changes of filters required. In addition, the gas can mitigate fume formation and accelerate printing times, making the printing process safer and lowering the cost per part. It is alloy agnostic and ideal for additive manufacturing of lattice structures.
With such advancements in additive manufacturing technologies, nitinol will not only enable the future of medical procedures to become less invasive, but it will also open up possibilities to manufacture leading-edge products and components that have previously been out of reach.
Related Content
3D Printed Cold Plate for an Electric Race Car: The Cool Parts Show #51
An unconventional lattice design and biomimicry are key to the performance of this fluid-cooled heat exchanger for a battery-powered race car.
Read MoreHow to Build 10,000+ Shot Molds in Hours
Rapid tooling isn’t so rapid when it takes days to 3D print a metal mold, and then you still must machine it to reach the necessary tolerances. With Nexa3D’s polymer process you can print a mold in hours that is prototype or production ready and can last for more than 10,000 shots.
Read MoreVulcanForms Is Forging a New Model for Large-Scale Production (and It's More Than 3D Printing)
The MIT spinout leverages proprietary high-power laser powder bed fusion alongside machining in the context of digitized, cost-effective and “maniacally focused” production.
Read MoreAM 101: What Is Binder Jetting? (Includes Video)
Binder jetting requires no support structures, is accurate and repeatable, and is said to eliminate dimensional distortion problems common in some high-heat 3D technologies. Here is a look at how binder jetting works and its benefits for additive manufacturing.
Read MoreRead Next
3D Printed Nitinol Opens New Possibilities for Arterial Stents
Researchers at Australia’s national science agency, CSIRO, have created the first self-expanding nitinol stents made via powder bed fusion. The project points to more opportunities with this shape memory alloy.
Read MoreWhat Effect Does Gas Flow Have in Metal Additive Manufacturing?
Sintavia has partnered with one of the world’s leading providers of industrial gases in order to understand and improve metal AM’s most significant process variable.
Read MoreInert Gas in Metal 3D Printing, Materials and Postprocessing
High-end metal additive manufacturing relies on the perfect gas composition to create products that meet the required material properties. But gas such as argon is also key to metal powder production, storage and postprocessing.
Read More